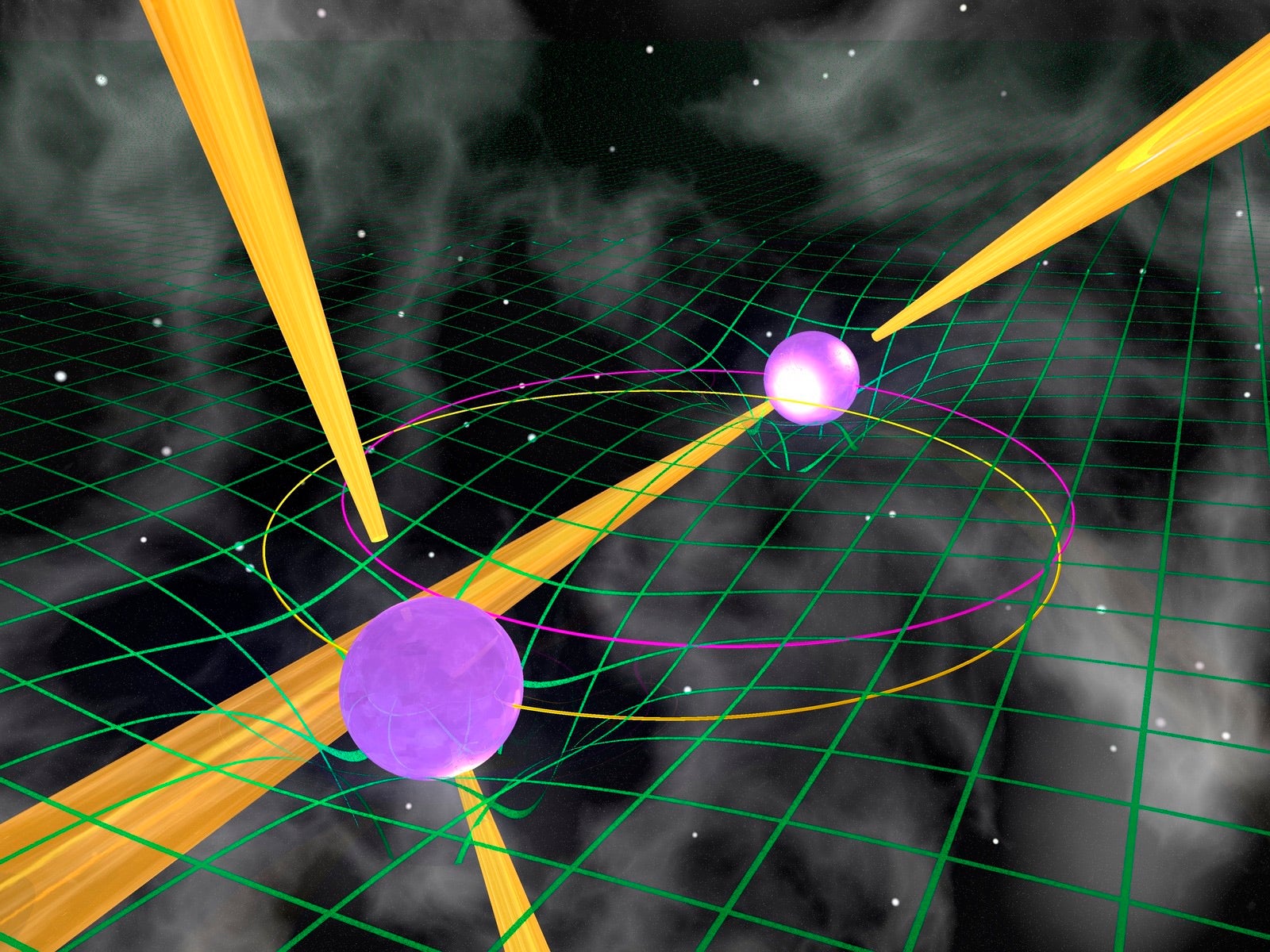
Fifty years ago, an unassuming bit of “scruff” appeared in Jocelyn Bell Burnell’s radio telescope data. Although barely noticeable at first, the scruff quickly led to two Nobel Prizes, provided evidence that Albert Einstein’s general relativity was right, rode on humanity’s first interstellar vehicle, and became the inspiration for a watch, a car, and an album cover.
The objects revealed by the scruff – dubbed “pulsars” – turned out to be smaller than a city but more powerful than the Sun. And if everything goes right, they soon will help us detect the most colossal events in the cosmos and even help us navigate to the stars.
Despite its meteoric rise to fame, the pulsar had an unpromising start. In July 1967, Bell Burnell noticed a quarter-inch-wide radio signal barely rising above the background noise on the recordings. It would have been easy for anyone else to ignore, but Bell Burnell had almost single-handedly strung 120 miles of wire to create the 4.5-acre radio telescope at Mullard Radio Astronomy Observatory near Cambridge, England, making her fast friends with everything it had ever picked up. She was not about to let anything escape her attention, no matter how scruffy.
Fortunately, the scruff showed up more than once. In fact, as she pored over the miles of charts, the keen-eyed graduate student spotted the signal on about 10 percent of the printouts, arriving four minutes earlier each day. Keeping time with the stars, the source definitely was not terrestrial. But what was it?
She persuaded her Ph.D. adviser, Antony Hewish, to speed up the paper feed so she could better scrutinize the odd signals. For weeks, miles of charts streamed through. As the piles of paper grew, so did Hewish’s frustration. Finally, on November 28, 1967, as they were about to pull the plug on the search, the signal returned.
Now, instead of a bit of scribbly fuzz, a series of regularly spaced shallow bumps appeared, each separated from its neighbors by 1.3373 seconds. These precisely timed, rapid radio blips telegraphed information about uncharted astrophysical territory. Because known stars could not change brightness so rapidly, the scientists knew they were looking at an unfathomably dense, small object. Or did they dare suggest, could it be something artificial?
LGM or not?
Although Bell Burnell and Hewish agreed that the latter explanation was highly unlikely, unusual signals are a siren song to astronomers. Despite healthy skepticism, most people are open to the possibility that someone, or something, in this vast universe might try to make contact. And so Bell Burnell gave the object the tongue-in-cheek nickname “LGM-1,” for “Little Green Men.”
The next step was to look for Doppler shifts, telltale changes in the signal’s wavelength that let astronomers know an emitting object’s comings and goings. These new data would let them know if the signal had been broadcast from a planet orbiting a star. Before that investigation even got off the ground, however, another similarly regular signal joined LGM-1. Then another. And another.
At that point, Bell Burnell recalls, they gave up on the idea of aliens. “It was extremely unlikely that there would be four separate lots of Little Green Men, all, at the same time, signaling to the inconspicuous planet Earth using a stupid frequency and a daft technique,” she said.
Although the signal proved not to be an extraterrestrial greeting, it was a tremendous astronomical discovery in its own right. The researchers soon found out that whatever it was, it kept time to better than 1 part in 10 million, either rotating or pulsating (they weren’t sure which) more than 60,000 times per day. On top of this, it was emitting enough power across interstellar distances to be detected even by 1967 instruments.
The neutron star connection
This sort of activity required a phenomenal source of energy. Astronomers Fritz Zwicky and Walter Baade hinted at one back in 1934, when they hypothesized that an exploding massive star — they called it a “supernova” — might leave behind a dense core composed mainly of neutrons.
It was a wild idea with seemingly little chance of verification. How would anyone ever detect a city-sized ball of neutrons, even one at a temperature of a million degrees? For the most part, astronomers ignored Zwicky and Baade’s prescient paper and quietly assumed that massive stars died by blowing themselves to smithereens.
Then came LGM-1, which soon became CP 1919+21 and ultimately PSR B1919+21. If the object were physically pulsing, growing and shrinking, Bell Burnell and Hewish computed a density equivalent to a mountain squeezed into a thimble: 10 trillion grams per cubic centimeter, the same density as a ball of neutrons.
Since it was a pulsating signal from a stellar source, science journalist Anthony Michaelis christened the object a “pulsar” in 1968. Although scientists were still several months from understanding whether the object was pulsating or rotating, the moniker stuck.
It was admittedly a catchy name. Less than three years after Bell Burnell’s discovery, the Hamilton Watch Co. began developing the Pulsar watch, capitalizing on nature’s seemingly perfect timekeepers. The first Nissan Pulsar, which was — what else? — a compact car, rolled off the assembly line in 1978.
In 1979, pulsars slipped effortlessly and largely unrecognized into popular culture. Next to the entry in the 1977 edition of The Cambridge Encyclopedia of Astronomy was an image of successive stacked pulse profiles from PSR B1919+21. The simple but enigmatic portrait of squiggles might have remained in that encyclopedia and in obscure journal articles if it hadn’t caught the eye of Peter Saville, who decided that it would make the perfect cover art for Joy Division’s 1979 album Unknown Pleasures. These days, plenty of people recognize the image that’s been featured on everything from T-shirts to tattoos, but few realize it represents the first pulsar discovered.
So what exactly are these objects that have captured the attention of astronomers, watchmakers, car companies, and album art designers? The simplest description is that they are rapidly rotating, highly magnetized, city-sized, collapsed cores of dead, high-mass stars — the neutron stars Zwicky and Baade proposed.
But this portrayal utterly fails to convey the bizarre and extreme character of a pulsar. Nature crams up to three times the mass of the Sun into its tiny volume, making its surface gravity second only to that of a black hole. Its magnetic field strength can be a quadrillion times Earth’s, far exceeding anything scientists can create in a laboratory.
This mega-magnetism whips up charged particles in the vicinity, causing them to swarm around the magnetic poles and emit beams of intense radiation. These beams become a sort of lighthouse beacon, sweeping across the universe as the neutron star spins. On the off chance that Earth happens to lie in the beam’s path, we see a quick blip with every rotation.
Pulsars are laboratories of extreme physics, and their discovery opened up an entirely new field. The find was so significant that just seven years later, Hewish would share the Nobel Prize in Physics “for his decisive role in the discovery of pulsars.” The exclusion of Bell Burnell, who treated even the smallest, most unusual bit of data as significant, has been hotly debated ever since.
Astronomers estimate that up to a billion massive-star remnants should exist in the Milky Way, but only a million or so are pulsars. So far, only about 2,300 have turned up. Pulsars aren’t permanent, after all. As the rotation, magnetic field, and local supply of electrons peter out over a few million years, so does the lighthouse beam. During a human lifetime, however, a pulsar’s timing is typically as precise as an atomic clock’s, changing by about a billionth of a second per year.
The presence of a stellar companion, though, can wind them up and turn them into even better timing devices. In 1982, astronomers discovered the first millisecond pulsars, objects whirling around at more than 100 times per second. The current record belongs to PSR J1748–2446ad, which rotates 716 times per second. Not merely curiosities, these speed demons may help future astronauts find their way.
An early warning of pulsars
U.S. Air Force Staff Sgt. Charles Schisler knew something was odd in the radar signals he was monitoring. It was summer 1967, and the signal had been showing up for weeks, keeping time with the stars by rising four minutes earlier each night.
Unfortunately, his employer wasn’t at all interested in this sort of signal. The Ballistic Missile Early Warning System at Clear Air Force Station in Alaska was built to detect incoming warheads, not pulsars. As a result, Schisler wasn’t at liberty to announce his discovery or do any formal follow-up observations.
It wasn’t until 40 years later that information from the Early Warning System became declassified, and Schisler felt comfortable revealing that he had observed not just one pulsar, but a dozen or so. Even then, Schisler was quick to point out that his detections never hinted at the pulsing nature of these sources, a key to understanding the objects responsible for the signals. That honor still goes to Jocelyn Bell Burnell. — C. R. J.
Toward a pulsar GPS
Astronomers hatched the idea to use pulsars as navigation tools on the heels of their discovery, but in 1972, it literally was etched in gold. As scientists were putting the finishing touches on the Pioneer 10 spacecraft for its journey through the solar system and beyond, they wondered if they could convey the craft’s “home” to any extraterrestrials it might come across. If only there were some way to indicate our position relative to objects with precisely known properties, like a sort of galactic GPS.
“Pulsars are the obvious answer,” Carl Sagan declared. And with just three weeks left to design and etch the gold plaque that would ultimately be affixed to Pioneer 10 and its twin, Pioneer 11, he and Frank Drake created a map of the Sun relative to 14 pulsars. They also included a code indicating universal constants that intelligent aliens should be able to decipher. With the map and the spin-down rate of each pulsar, extraterrestrials would know not only where we are, but also when we launched the probe.
Maybe. “The data for one pulsar on the plaque is wrong, and so the aliens would need to sort that out!” jokes pulsar researcher George Hobbs at Australia’s Commonwealth Scientific and Industrial Research Organization.
What about us? Could we ever use pulsar GPS as we leave the safety of Earth? Our current GPS, which uses Earth-orbiting satellites, obviously wouldn’t help us navigate to Mars, much less to other star systems. Looking to the future, Hobbs and his colleagues have explored the feasibility of using pulsars as navigation tools.
The idea is straightforward in principle. On Earth, we know when to expect pulses from pulsars. And because light takes time to travel, those arrival times depend on our exact location. Complicating matters is the fact that pulsars, well, pulse — frequently. The universe hosts an infinite number of places where you can expect a pulse from a given pulsar at a particular time, but by observing several pulsars, you can quickly narrow the set of places you might be.
It takes a bit of computational gymnastics to settle on the right one, but Hobbs and his colleague You Xiaopeng have performed those acrobatics with data from millisecond pulsars and successfully pinpointed the location of the 64-meter Parkes radio telescope in Australia to within 0.6 mile (1 kilometer). They even confirmed that Earth revolves around the Sun, just in case anyone was still on the fence about that.
“The main challenge is being able to detect pulsars without a huge telescope,” says Hobbs. “Of course, you’re not going to launch the Parkes telescope into space!”
Even if you did, it wouldn’t be of much use. A single radio dish can observe only one pulsar at a time, but, as is the case with terrestrial GPS, you need simultaneous information from several different clocks. A single X-ray telescope might do the trick, however, and several teams around the globe have jumped on the X-ray pulsar navigation bandwagon.
“The Europeans seem to have a crazy package to see if they can navigate aircraft [with pulsars],” says Hobbs. “And the Americans and Chinese are both undergoing research into deep-space navigation with pulsars.”
A time for pulsars
Pulsar timing should prove useful for more than navigation. When he’s not wrestling with issues of pulsar GPS, Hobbs and others are looking to these neutron stars to help us listen in on the most gargantuan cosmic events.
We can thank Einstein for the idea that our universe is an interwoven tapestry of matter, space, and time. According to his theory of general relativity, when matter moves, the rest of the tapestry ripples predictably in response. On September 14, 2015, the Laser Interferometry Gravitational-wave Observatory (LIGO) directly detected gravitational waves for the first time. Two black holes, each about 30 times the mass of the Sun, collided over a billion years ago, causing space to literally (and measurably) contract and expand.
But many people aren’t aware that pulsars have been whispering rumors about gravitational waves for decades, giving astronomers confidence that they would ultimately detect them directly. In 1974, Russell Hulse and Joseph Taylor Jr. at the University of Massachusetts, Amherst, announced the discovery of a pulsar in orbit around another non-pulsing neutron star. It was the first of its kind: two extreme objects locked in a frantic dance, whirling around each other every 7.75 hours. The system pulls relentlessly at the fabric of the universe, which, in turn, saps energy from the pair. The lost energy spreads into the universe as gravitational waves.
The scenario might sound vaguely familiar. This binary pulsar — PSR B1913+16 — is performing a small-scale version of the tarantella executed by LIGO’s famed colliding black holes. Long-term observations show that PSR B1913+16’s orbit is shrinking by about 3.2 millimeters per circuit, with the two neutron stars scheduled to collide in just 300 million years. The system provides researchers with an extreme gravitational laboratory, one for which Hulse and Taylor received the 1993 Nobel Prize in Physics.
Pulsars probe gravitational waves
Pulsars can do more than merely insinuate the existence of gravitational waves, however. Because rotating neutron stars make exquisite clocks, they can be the endpoints of a galactic LIGO setup. LIGO detects passing gravitational waves by measuring how they compress and stretch the instrument’s arms. These waves should do the same to the space between pulsars and Earth. Astronomers would then measure subtle variations in the pulse periods, and those changes would depend on the pulsar’s direction. LIGO has only four observational arms: two in Livingston, Louisiana, and two in Hanford, Washington. With pulsars, astronomers can have as many observational arms as there are observed pulsars.
It’s an elegant and simple plan, one that officially got underway more than a decade ago with the creation of the International Pulsar Timing Array (IPTA). But IPTA is after much bigger quarry than the stellar-mass black hole mergers seen by LIGO. It’s trying to listen in on collisions of supermassive black holes. Paradoxically, this task is even more challenging than detecting the mergers of star-sized black holes.
Paul Lasky, a member of both gravitational wave teams, explains the process: “When two galaxies collide, the black holes in their cores will eventually sink to the center of the merged galaxy, and those two black holes will eventually merge themselves.” It would seem that the sheer magnitude of such an event would make it easy to detect, but the resulting gravitational waves would have a phenomenally low frequency.
“The period of the waves is approximately one to 10 years,” says Lasky. “This means that you need to observe for that amount of time to actually detect a single period.” So even with a decade of observing under their belts, astronomers aren’t too concerned that IPTA has yet to detect a merger of supermassive black holes.
There is one puzzling gap in IPTA’s results, however. If you throw a large rock into a perfectly calm pond, you will see symmetric ripples radiate from the entry point. But the universe has thrown lots of rocks of varying sizes into the cosmic fabric over billions of years, and that should create a jumble of interacting ripples, or what scientists call the stochastic background. “The fact that we have not seen this stochastic background in more than a decade of observations is slightly at odds with theoretical predictions,” says Lasky.
Still, with the resounding success of LIGO, gravitational wave scientists now can look more deeply into phenomena that until recently were on the fringe of observational astronomy, just as pulsars were a half-century ago. And there is every reason to believe that in the next 50 years, pulsars will continue to play a starring role in our understanding of the universe.
Not bad for a bit of scruff.
The pulsar hound of Parkes
Although it was up and running by 1961, the 64-meter Parkes radio telescope in New South Wales, Australia, was not the first to find evidence of pulsars, or even ferret out the first millisecond pulsar. In fact, the radio scope, nicknamed “The Dish,” came quite late to the pulsar game.
The telescope — one of the antennas that received the Apollo 11 TV transmissions from the Moon — amassed just eight pulsar discoveries by 1973 and added only about 300 more over the next quarter-century. But once scientists installed a new receiver in 1997 that provided much wider sky coverage, The Dish leapt into action. It would detect more than 100 pulsars per year for the next seven years, outpacing all other radio observatories put together.
The Dish’s most significant discovery arguably came in 2004, when it revealed a double pulsar system. A step beyond Hulse and Taylor’s pulsar-neutron star binary, the double pulsar allows astronomers to probe Einstein’s general relativity further while exploring an eclipsing pulsar system.
All told, The Dish has discovered about two-thirds of the 2,300 or so known pulsars. In addition, it plays a leading role in the International Pulsar Timing Array, the development of pulsar GPS, and bringing pulsar science to a new generation through PULSE@Parkes, an educational program for high school students. — C. R. J.