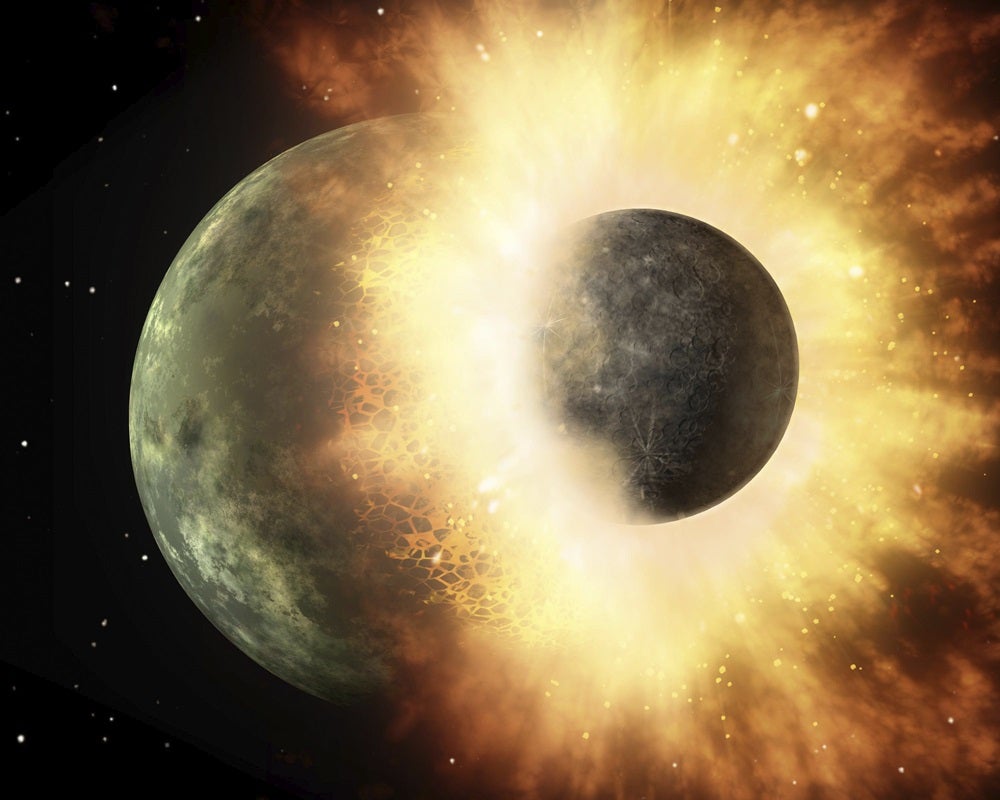
T he Moon has always beckoned. Long before our ancestors realized “wandering stars” were actually planets sharing the solar system with Earth, they recognized the Moon was a sort of sibling to our planet. And surely one of the first big questions to arise was: How did the Moon come to be?
Fifty years ago, humans accomplished one of our greatest feats of exploration when we set foot on the Moon. The Apollo program has been recognized as a political and technological triumph, but less widely appreciated is the scientific windfall brought by the valuable lunar samples Apollo astronauts returned to Earth. These relics have ultimately proven vital to answering the age-old question of how the Moon formed.
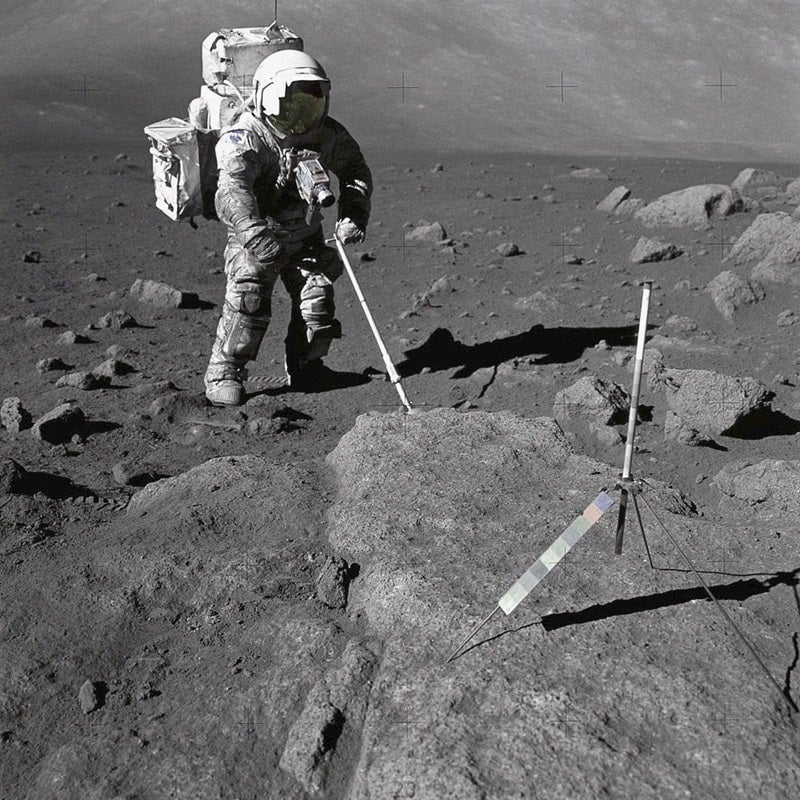
Apollo rocks reveal the Moon’s past
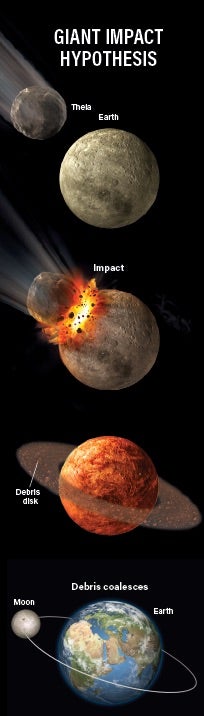
Our planet has largely erased the record of its ancient past, thanks to a continual reshaping of its surface through geological activity. But the Moon is essentially dormant, so its heavily cratered surface preserves a record of solar system events going back billions of years. Thus, the Moon also opens a window into our planet’s primordial history.
One of the main goals of the Apollo program was to distinguish among the then-leading theories about how the Moon formed: capture, co-formation, and fission. The capture theory posited the Moon formed independently from Earth, only to be caught later by our planet during a fortuitous close flyby. The co-formation theory, however, envisioned the Moon grew alongside Earth, with the pair accumulating mass from the same source of material. The third model, fission, proposed ancient Earth rotated so rapidly that it became unstable, developing a bloated midsection and shedding material from its equator that would eventually become the Moon.
Apollo’s cache of lunar samples and data introduced researchers to tantalizing new clues and constraints for these three models. For instance, measuring the age of the oldest Apollo samples showed that the Moon must have formed some 4.5 billion years ago, only 60 million years or so after the first grains in our solar system condensed. This means the Moon came to be during the same early epoch that saw the birth of the planets.
From remote measurements of the Moon’s mass and radius, researchers also know its density is anomalously low, indicating it lacks iron. While about 30 percent of Earth’s mass is trapped in its iron-rich core, the Moon’s core accounts for only a few percent of its total mass. Despite this substantial difference in iron, Apollo samples later revealed that mantle rocks from the Moon and Earth have remarkably similar concentrations of oxygen. And because these lunar and terrestrial rocks differ significantly from meteorites originating from Mars or the asteroid belt, it shows the Moon and Earth’s mantle share a past connection. Additionally, compared with Earth, lunar rocks are more depleted in so-called volatile elements — those that vaporize easily upon heating — which hints that the Moon formed at high temperatures.
Finally, researchers know that tidal interactions forced the Moon to spiral outward over time, which in turn caused Earth to spin more slowly. This implies the Moon formed much closer to Earth than it is now. Precise measurements of the Moon’s position using surface reflectors placed during the Apollo program subsequently confirmed this, verifying the Moon’s orbit expands by about 1.5 inches (3.8 centimeters) each year.
Giant Impact Hypothesis
As is not uncommon in science, the Apollo data, which was originally intended to test existing theories, instead inspired a new one. In the mid-1970s, researchers proposed the Giant Impact Hypothesis. The novel scenario envisioned that at the end of its formation, Earth collided with another planet-sized body. This produced a great deal of debris in Earth’s orbit, which in turn coalesced into the Moon. The impacting planet would later be named Theia, in honor of the Greek goddess who was the mother of the Moon.
The new impact theory seemed to reconcile multiple lines of evidence. If the material that formed the Moon originated from the outer layers of Earth and Theia, rather than from their cores, an iron-poor Moon like we observe would naturally result. A giant impact that struck Earth obliquely could also account for Earth’s rapid initial spin. Finally, the enormous energy released during such an impact would vaporize a substantial portion of the ejecta, accounting for the Moon’s lack of volatile materials.
The scientific community was initially skeptical of this new model. The impact hypothesis was critiqued as being a contrived, ad hoc solution that might represent an extremely unlikely event. But at the same time, work on the competing models proved increasingly unsatisfying.
The energy dissipation needed to capture the Moon intact during a close flyby seemed implausible, if not impossible. Models of the Moon’s co-formation alongside Earth struggled to explain why the Moon would have a vastly different proportion of iron. Additionally, the current angular momentum of the Earth-Moon system is too low to be explained by a rotationally unstable Earth that flung off enough material to form the Moon. Although researchers carried out little quantitative work on the giant impact model at first, it eventually emerged as the most promising theory during a mid-1980s conference on lunar origin, largely due to the weaknesses of competing theories.
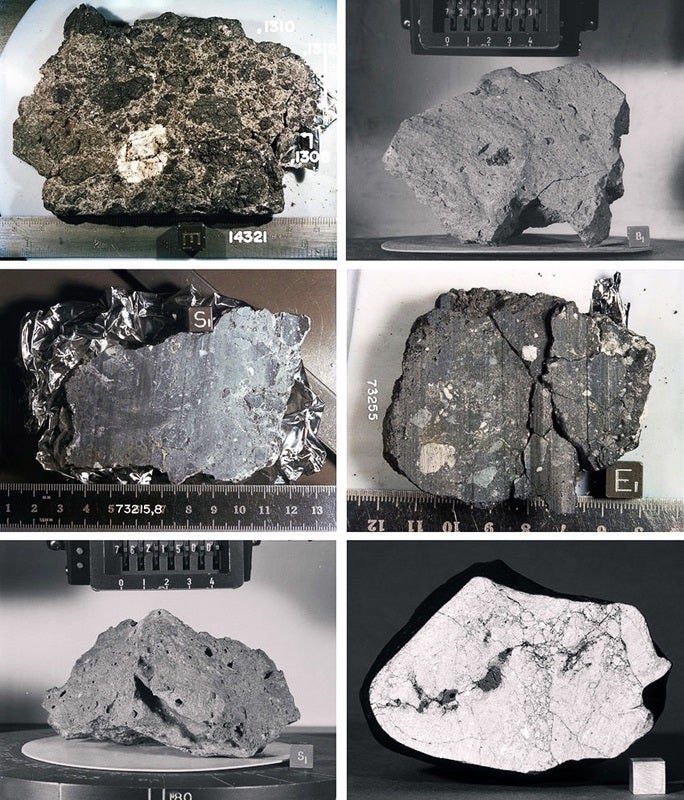
But could a giant impact really produce the Moon? The answer to this question was not obvious at the time. From basic physics, scientists knew that ejecta launched from a spherical planet should either escape entirely into space or plummet back to the planet’s surface. The ejected material should not simply enter into a stable orbit around the planet. However, a large enough impact — one by a body roughly the size of the target itself — distorts the shape of the impacted planet, altering its gravitational interactions with the ejecta.
Additionally, researchers knew partially vaporized material can get its own acceleration with the help of escaping gas, which changes the material’s trajectory. However, assessing the effects of this scenario required a new generation of computer simulations at a scale never before modeled. With then-available technology, such simulations were extremely challenging, but researchers eventually showed that giant impacts could produce orbiting material that might assemble itself into the Moon.
Thanks to vast computational improvements, researchers had identified by the early 2000s what would later become known as the “canonical” impact theory: a low-velocity collision at about a 45-degree angle by Theia, which had a mass similar to that of Mars. Such an impact would produce an iron-depleted disk of material massive enough to form the Moon, while simultaneously leading to a five-hour day for Earth. Then, over billions of years, tidal interactions transferred angular momentum to the Moon, which gradually pushed the Moon outward and slowed Earth’s spin. This fits well both with Earth’s current 24-hour day, as well as the present orbital distance of the Moon.
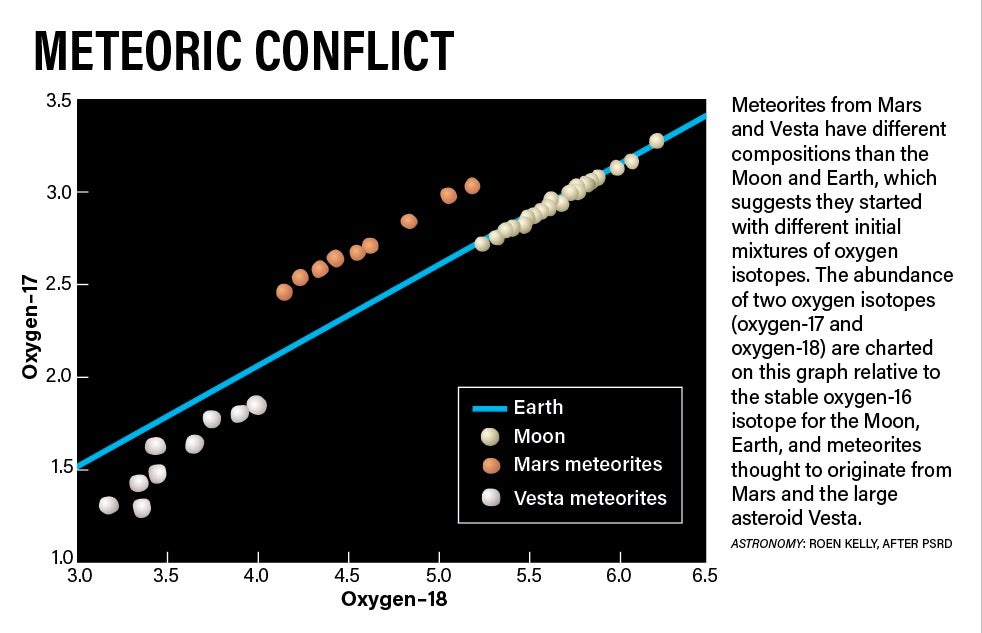
Lingering questions
If the Moon were like other astronomical bodies, for which we typically have only remote observations, at this point we would have likely declared the origin story of the Moon solved. In this case, however, we have physical samples from both the Moon and Earth that we can compare. Explaining the chemical relationship of those samples has proven to be the biggest challenge to the Giant Impact Hypothesis, inspiring a flurry of work over the past decade investigating how exactly the Moon came to be.
The conundrum is this: In most giant, disk-forming impacts like those described above, it is mainly material from the outer portions of Theia that are slung into Earth orbit. But we cannot know for certain what Theia’s composition was when it impacted Earth. If Theia, like Mars or main-belt asteroids, were made of different material than Earth, then ejecta coming from Theia should have a different composition than our planet.
Instead, data derived from Apollo lunar samples increasingly show that the Moon and Earth are almost chemically indistinguishable, not just for oxygen, but for many other elements, too. They have similar isotopes, or variations, of the same elements. Solving this isotopic crisis requires explaining how the collision of two independently formed planets, each with its own distinct history and composition, could have produced two such indistinguishable offspring.
One feasible explanation is that Theia did have an Earth-like composition, perhaps due to both bodies forming from shared material at a similar distance from the Sun. In fact, there is evidence that the impactors that delivered the final 40 percent of Earth’s mass all had the same composition, indicating they formed from the same stuff. However, new analyses of lunar samples highlight one likeness between Earth and the Moon that doesn’t exactly add up, and it involves the element tungsten.
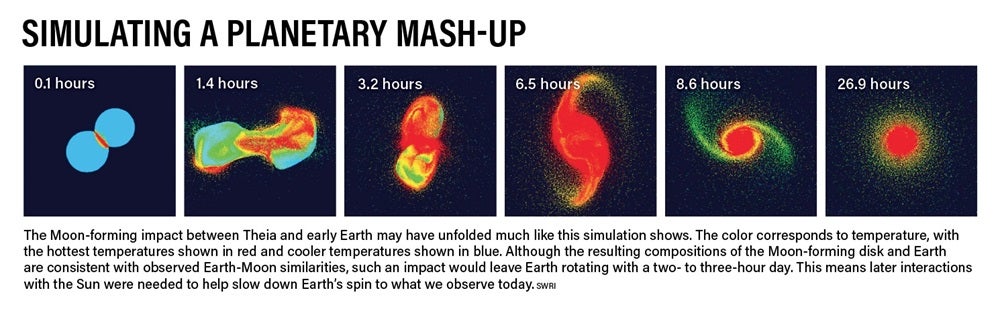
ungsten is particularly useful for understanding a planet’s origin for two reasons: It tends to be incorporated into a planet’s metallic core as it forms, and one isotope, or flavor, of tungsten is produced through the radioactive decay of the element hafnium, which was prevalent only during the first roughly 60 million years of the solar system’s history. Unlike tungsten, hafnium is generally not incorporated into a planet’s core, and instead remains within its mantle. Assuming a planet’s core formed during the first 60 million years — as was likely true for both Theia and Earth — the abundance of hafnium and, thus, the particular isotope of tungsten it produces in its mantle, is extremely sensitive to the timing of its core’s formation. In other words, even if Theia had been Earth-like in elements like oxygen by virtue of forming near Earth, an additional coincidence in the timing of their formation would be needed to produce the observed Earth-Moon tungsten match. Current estimates suggest such a coincidence would have been highly improbable.
An alternative scenario envisions that the giant impact produced a Moon-forming disk that was chemically distinct from Earth at first. Then, vaporized portions of Earth mixed together with vapor in the disk, equalizing their compositions. In this equilibration model, the mixing of material essentially erased the chemical signature of Theia in the Moon-forming disk.
Equilibration is an appealing process because it could account for why Earth and the Moon show similarities across many elements, including tungsten. However, such mixing must have occurred rapidly, because it likely took the Moon only a few hundred years to form from the disk. Whether such efficient mixing occurred over such a short time period remains uncertain.
Variations on a theory
In 2012, researchers made an important discovery. They showed that certain gravitational interactions with the Sun could have allowed Earth to slow its rotation by a factor of two or more through the siphoning of angular momentum from Earth’s spin into its orbit around the Sun. If this is possible, it means Earth’s rotation rate just after the Moon formed could have been even faster than previously assumed — spinning about once every two hours instead of every five hours. This would indicate an even more forceful impact with Theia occurred.
Researchers have proposed a variety of high-angular-momentum impacts that could produce such rapidly rotating Earths, including some that lead to a disk and planet with nearly equal mixtures of material from both Theia and early Earth. The exact slowdown needed to explain a larger, higher-energy impact, however, would require a narrow range of parameters that are, as yet, still quite uncertain. This makes the scenario’s overall likelihood unclear.
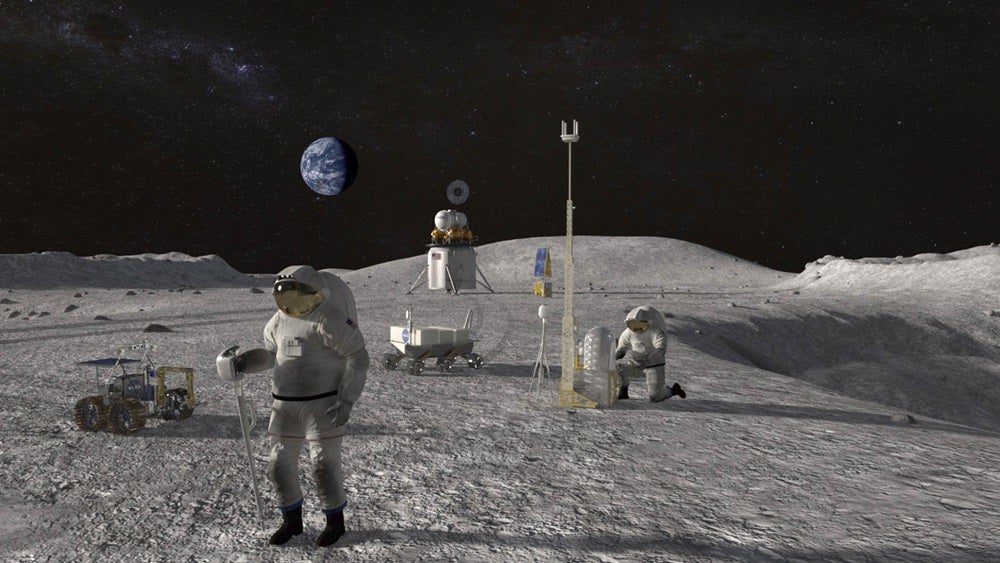
But what if the Moon were the product of multiple impacts, rather than just one? Recent alternative models consider the Moon formed via tens of smaller impacts with Earth, rather than a single, giant impact. In this scenario, a relatively small impact creates a “moonlet” whose orbit spirals outward. A later impact produces another moonlet, and its outward migration could cause it to merge with the first. A full-sized Moon built up by many smaller impactors with a range of compositions is more likely to end up with an Earth-like composition than a Moon produced by a single impact. However, the problem with this theory is that moonlets formed by different impacts don’t necessarily merge. Instead, it’s more likely that such moonlets would get ejected from orbit or eventually collide with Earth.
A final question is whether lunar impact simulations have considered all important aspects of a Moon-forming collision. Prior studies have generally found similar outcomes even when different conditions and computational approaches are adopted. However, new research proposes that if Earth’s mantle was molten at the time of the giant impact — due to heating from a recent prior impact — then much more terrestrial material could be ejected into space, leading to a more Earth-like disk, even for a giant impact scenario.
Where do we go from here?
Thus, we find lunar origin models at a crossroads of sorts. On one hand, many once-uncertain aspects of the Giant Impact Hypothesis have been validated. Current planet-formation models predict that giant impacts were commonplace in the inner solar system as Earth grew. Thousands of increasingly sophisticated simulations have established that many (if not most) giant impact scenarios would produce disks and moons. The Moon’s lack of iron, which is difficult to explain in competing models like intact capture, results naturally from a large impact. This is because the material that coalesced into the Moon comes from the outer mantles of the colliding bodies rather than from their iron-rich cores.
However, explaining other characteristics still poses a difficult challenge. Specifically, it’s hard to account for the ever-growing list of elemental similarities between Earth and the Moon, as revealed by lunar samples. One would expect the collision of two planets to have left some trace of their compositional differences, and yet — at least based on current data — such differences are not evident.
Researchers have proposed many new, creative explanations for how an impact (or impacts) could have produced a Moon so chemically similar to Earth. However, the new ideas impose additional constraints. Thus, the impact theory still grapples with the question it faced nearly half a century ago: Would such an event have been likely, or does it require the Moon to be the product of a very unusual event?
Making headway depends on developments across several fronts. Researchers will need to employ next-generation models to link the varied origin scenarios to predict the Moon’s properties, which will then be tested by comparing them to observations.
Fortunately, the U.S. and other space-faring countries are planning upcoming Moon missions that aim to provide crucial new constraints. For example, new lunar samples may more fully reveal the Moon’s composition at depth. Improved measurements of lunar seismic activity and heat flow may better constrain the Moon’s internal composition and initial thermal state.
Ultimately, we will continue to pursue the answer for how our Moon came to be, not only so we can understand the history of our home world, but more generally, so we can learn what our nearest cosmic neighbor can teach us about the formation and evolution of inner planets — both in our solar system and beyond.