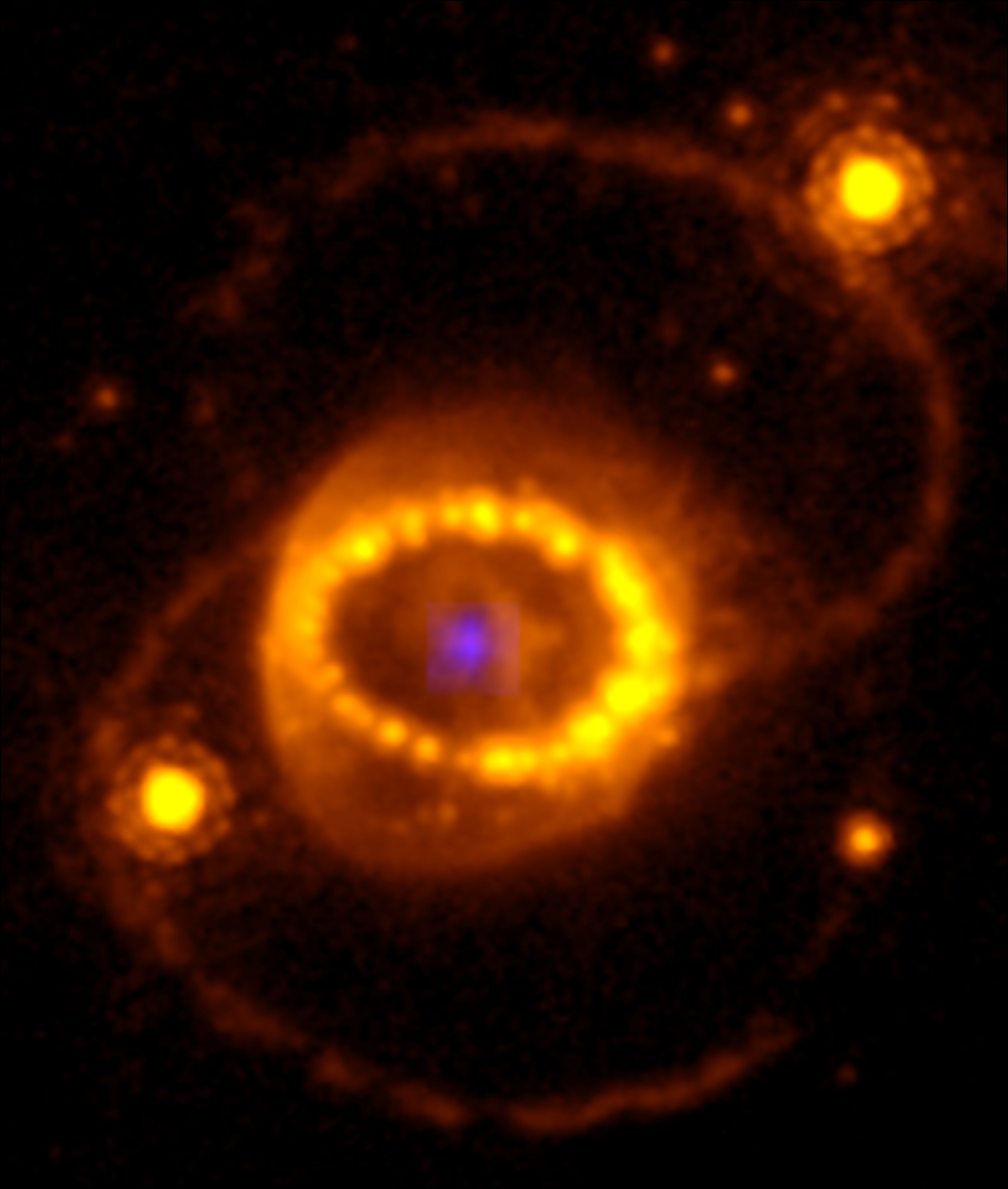
In 1987, astronomers had an incredible front-row seat to a cosmic event that maybe isn’t rare, but rarely happens near us.
Just 168,000 light-years away, on February 23 of that year, a star in the Large Magellanic Cloud, the largest satellite galaxy of the Milky Way, exploded. This happened when the star’s core collapsed into a dense object and expelled the outer gas layers, giving astronomers an iconic show.
As the closest supernova that has happened in our lifetimes, Supernova 1987A (SN 1987A) has been studied, scrutinized and dissected by some of the largest observatories, giving us a chance to do forensics on a dead star from the moment of demise to its 37-year aftermath.
And now, thanks to the James Webb Space Telescope (JWST), we finally know what happened to the core. New research published in Science describes how astronomers used the infrared capabilities of the telescope to peer behind the dust cloud that remains where a star once was. There, they found a neutron star, a dense ball of matter about the size of a city but containing a mass one or two times that of the Sun.
“The observations are so much better than anything which has been done, especially in this part of the spectrum, but the details we see with this image are so much better than you can do even with the Hubble telescope,” says study lead author Claes Fransson of Stockholm University.
When stars go supernova, one of two outcomes happens. The core either becomes a neutron star or it collapses into a black hole. The star’s fate depends on its mass as well as the mass of the core. A core above about 2.2 times the Sun’s mass is destined to become a black hole, while a star below that threshold becomes a neutron star. (Some neutron stars rotate in such a way that a beam of light is pointed at Earth, visible in radio frequencies, but Fransson says there’s no indication right now that SN 1987A is a pulsar.) The star that formed SN 1987A had been observed before, so we had a relative idea of its size — somewhere between 15 and 20 times the mass of the Sun.
This is big, but it’s not the biggest a star can get, so models erred on the side of predicting a neutron star. But while there were a few hints along the way that this was likely the case, the JWST observations managed to image the neutron star itself. This resulted after observations published last year showed the shape of the ejecta coming out from the core.
JWST will be able to track the star’s evolution over time, a feat it has already been working on. Frannson alludes to observations made last autumn that have not yet been published, which saw the density of material ejected by the supernova thin out a little. As this takes place, the neutron star will become easier to study.
“This intervening material in the center is getting thinner and thinner, so we see the center better and better, so that’s a very strong reason for continuing the observations,” Fransson says.
The telescope’s onboard spectrometers were also used on the area around the neutron star to learn more about the star’s current composition. Although the data are new and have not been fully analyzed, a cursory glance reveals that “the details and the spectrum are much better than we had with the first set of observations, so that gives us completely new ways of looking at it,” Fransson says.
All these observations from JWST — as well as observations from other observatories — help us complete the picture of what happened 37 years ago. But future observations will help build a timeline of what happens in a star system for the first few decades after it explodes, and will help astronomers know what to look for the next time a nearby supernova explodes.