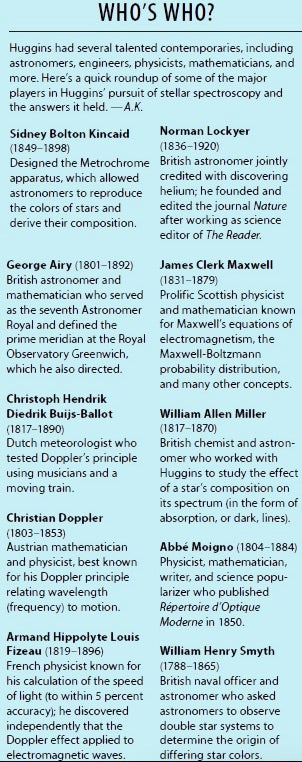
For most of human history, observers envisioned the night sky as a sphere studded with fixed stars. It is easy to understand why. Our lives are too short and our unaided senses too limited to detect any stellar motion. In 1718, Edmond Halley discerned the “particular motion” of three stars across the sky based on careful comparison of ancient and contemporary star maps. But stars offer none of the usual cues we might use to tell if they also moved along our line of sight.
That changed in 1868 when English astronomer William Huggins applied the spectroscope and Doppler’s principle to the problem. The impact on the theory and practice of astronomy was revolutionary. Within a generation, the questions astronomers could ask about the bodies they observed and the methods deemed appropriate to examine them changed in ways their predecessors could not have imagined.
Huggins’ name might be unfamiliar to modern ears, but the self-taught amateur astronomer was celebrated in his own lifetime as the father of astrophysics. Born in 1824, he sold his family’s London silk shop at the age of 30 to devote himself full time to his growing interest in astronomy. In 1856, he built a substantial observatory attached to his home and recorded his first observations in a bound notebook. In the early 1860s, he drew widespread acclaim for his pioneering work in celestial spectroscopy, the technique of splitting starlight into its constituent wavelengths. (For the details of this process, see “How does spectroscopy work?” on p. 47.) Huggins was among the first to compare stellar spectra directly with those of terrestrial elements, the first to observe emission lines in the spectra of nebulae, and the first to observe a nova’s spectrum.He designed a better way to compare the star and spark spectra simultaneously, but on March 6, he complained he was unsure of the comparison spectrum’s alignment: “Made the observation at least 20 times but without absolute certainty.”
On March 10, he cheerfully recorded that he was “almost certain after a great number of trials” that Sirius’ F line was shifted toward the red – only to have his confidence shaken two nights later when the star’s lines once again appeared to be shifted toward the blue, the opposite of his earlier “very satisfactory” observations.On March 30, he was relieved to see Sirius’ spectrum again shifted toward the red. “Taken in connection with the satisfactory result of [February] 24, it may be considered as certainly confirmatory of the observations on that night. I made numerous comparisons during an hour, almost always the same result.”By April 4, with Sirius too low in the sky, Huggins compiled his observations and submitted his paper to the Royal Society on April 23. It was titled “Further Observations on the Spectra of Some of the Stars and Nebulae, with an Attempt to Determine Therefrom Whether These Bodies are Moving towards or from the Earth.” Its tone is one of confidence and spirited adventure. He advertised the soundness of his measures and the theoretical foundation for his interpretation of them. He expressed satisfaction that he had resolved his instrumental problems through clever manipulation of instruments and enviable patience.Huggins does not say how many observations he actually made, or of those, how many were discarded or why. In his view, throwing out those he deemed unworthy was simply judicious weeding conducted to ensure the reliability of his measurements.Although Maxwell still drew on Fizeau’s 1848 lecture, he now acknowledged that if an individual spectral line of a known terrestrial element could be compared simultaneously with its stellar counterpart, even small differences in its position could be detected with the right instrument. Then, Doppler’s formula could be used to calculate the relative motion of the star and Earth along the line of sight.
Huggins had just purchased a new micrometer that he felt met the task’s demands for precision. On June 25, 1867, with the Moon at Last Quarter, he eagerly took aim at Arcturus (Alpha [α] Boötis), then perched high overhead in the evening sky. Unfortunately, poor conditions deprived him of “the steady distinction necessary” to detect “any motion towards or away from the earth,” he recorded in his observing notes.Today, measuring stellar radial velocity — mapping tiny shifts in spectral lines to determine the motion of a star relative to an observer — is a straightforward and routine procedure thanks to photography and precision instruments. Keep in mind that Huggins conducted his efforts entirely by eye. His notebook records help us understand how he handled the overwhelming challenges he faced at each step, and they bring into relief his skill in persuading his colleagues that he had, in fact, succeeded.Armed with a new and more highly dispersive spectroscope, Huggins tried again February 11, 1868, this time taking aim at Sirius (Alpha [α] Canis Majoris). He compared the star’s blue Fraunhofer F line, which appears due to the presence of hydrogen, with that of a hydrogen spark. He recorded: “Appeared to me very slightly more refrangible than line of [hydrogen].” In other words, Sirius’ line appeared shifted toward the blue end of the spectrum.
But on February 24, he found the star’s F line to be shifted toward the red!But visual observations could never satisfy astronomers’ need for precision. Instrumental instability and the faintness of a target star’s spectrum made it difficult for the human eye to detect the small displacements required for satisfactory measurements. After German astronomer Hermann Carl Vogel captured the first photograph of shifted stellar spectral lines in 1887, major observatories around the world became immersed in measuring the radial velocity of many different types of celestial bodies. They commissioned and purchased improved clock drives, state-of-the-art photographic equipment, and other precision instruments to aid in this work.
Private individuals could not afford such investments, and in time it became clear that Huggins had introduced a method that was well beyond his fellow amateurs’ reach. While Huggins cheered the progress of his professional colleagues, he moved on to other projects.Nevertheless, in daring to apply Doppler’s principle to astronomical inquiry, Huggins gave astronomy an elegant and reliable research tool of broad utility. More importantly, he successfully persuaded his contemporaries of the method’s potential. His early career as a silk merchant made him inclined to handle each and every innovation and discovery as a commodity to be packaged and sold, like an exotic bolt of cloth. He treated his colleagues like discriminating and sophisticated clientele, making every effort to instill in each a desire — even a need — to “buy” and use his newfangled methods.As we celebrate the sesquicentennial of Huggins’ introduction of Doppler’s principle into the astronomer’s toolkit, we commemorate the pioneering efforts of this gentleman scientist who participated with vigor and vision in the rise of astrophysics. We celebrate the risky choices he made as he moved from the periphery of scientific London toward its inner circle, choices that expose the dynamic and often uncertain process by which its boundaries of acceptable research were redefined during his lifetime. And we applaud his ability to persuade his colleagues of the theoretical authenticity, practical utility, and reproducibility of this method for measuring stellar motion in the line of sight.Testing the theory
Testing Doppler’s controversial ideas on star color and stellar motion may have been a back-burner project for Huggins that came to the fore only after he acquired instruments he believed were up to the demands of such a challenging effort. But that does not explain why he chose to launch the project when he did.
A possible trigger may have been a presentation on star color at the May 1867 meeting of the Royal Astronomical Society by Sidney Bolton Kincaid, a staunch adherent to Smyth’s plan to observe and record star colors. Kincaid described a new apparatus he called the Metrochrome. He had developed the device to replace previous methods of describing a star’s color in words or by a color illustration that could deteriorate over time. The Metrochrome used an incandescent platinum wire as an artificial star and an array of colored filters made of strictly prescribed chemical solutions as a means “by which the tints of fixed stars may be exactly recorded relatively to standards easily reproducible by any observer, with any kind of telescope, any number of years hence.”Kincaid’s talk would have thrilled Huggins. For one thing, the two had collaborated before. And Huggins was fascinated by gizmos. The idea behind the Metrochrome, its ingenious design, and its ease of use would have sparked his creative energies. It also would have refocused his attention on the old star-color question.
Did Kincaid reignite Huggins’ interest in star color and Doppler’s work? Or was it just a coincidence that Huggins chose this particular time to seek Maxwell’s advice on measuring the motion of stars in the line of sight? Whatever prompted Huggins to reconnect with Maxwell, it was a good move as he prepared to pursue that line of investigation. We can infer from Maxwell’s reply that both he and Huggins had given Doppler’s ideas some serious thought since May 1864, and had come to view those ideas as key to success in this endeavor.Huggins’ original query to Maxwell is lost. An excerpt from the reply he received on June 12, 1867, survives only because Huggins shrewdly included it in his 1868 paper. Maxwell’s letter fleshed out the theory and method underpinning Huggins’ novel investigation and placed authoritative weight behind his conclusions.At that time, members of the professional astronomical community saw the spectroscope’s celestial discoveries only as interesting distractions from the real task at hand, namely mapping the sky with precision to aid in terrestrial navigation and testing Newton’s laws. All this changed after Huggins introduced spectral analysis as a tool to detect and measure the motion of celestial bodies in three-dimensional space.
Solving star colors
To understand how Huggins was drawn to this line of investigation, we need to start with the enigma of star color. This characteristic had long intrigued observers. Did a star’s color have a real physical or chemical cause, was it more a matter of unavoidable instrumental distortion, or was it due to illusory individual perception? With no obvious means to resolve the issue with any certainty, it seemed that it might always remain a matter of creative speculation.
In 1844, English astronomer William Henry Smyth urged astronomers to methodically observe double star systems like Albireo (Beta [β] Cygni), which exhibit different, sometimes complementary colors. Jesuit astronomer Benedict Sestini took up Smyth’s challenge, and in 1850 he published a paper suggesting “variations in color may be owing to variations in stellar velocity.” Smyth expressed cautious interest in what he called “Sestini’s theory,” unaware that an explanation for star color had already been introduced nearly a decade earlier by Austrian mathematics professor Christian Doppler.Few people had heard of Doppler’s name or his work. His lack of notoriety was hardly surprising: When he presented his theory before the Royal Bohemian Society of Sciences in May 1842, only a handful of members were present. Doppler considered his theory as an extension to stellar aberration, the small annual shift in a star’s apparent position on the sky. While aberration is a natural consequence of both the finite speed of light and Earth’s orbital motion around the Sun in a plane perpendicular to a star’s incoming light, Doppler believed he had discovered something analogous resulting from motion parallel to its incoming light.Doppler was not an experimentalist. He based his theory on the mathematics of wave behavior, which describes how an observer’s perception of frequency will change if the wave source and the observer move toward or away from one another. Doppler pointed out that any detectable difference between a wave’s intrinsic frequency and the frequency perceived by an observer will make it possible to calculate the source’s relative speed of approach or recession. He applied this principle first to sound and then to light waves, claiming it could account for many previously unexplained astronomical phenomena, such as the colors observed in binary stars, as well as changes reported in the color and brightness of periodic variable stars and novae.In June 1845, Dutch meteorologist Christoph Hendrik Diedrik Buijs-Ballot devised a grand experiment to test Doppler’s ideas. During several trials, groups of musicians and musically trained observers switched roles as passengers on and bystanders alongside a noisy moving railway train. While the musicians struggled to sustain a steady monotone, the observers reported what they heard. Despite the tremendous challenge of executing these trials, Buijs-Ballot believed the reported changes in pitch matched Doppler’s predictions.
However, Buijs-Ballot was less sure about extending the theory to stars. The speed of light is nearly a million times faster than that of sound. He doubted any star moved fast enough for an observer to perceive a measurable difference in its appearance. Even if such a speedy star were found, he pointed out that Doppler was wrong to expect its motion to produce any hint of color. Buijs-Ballot assumed that any wavelengths that shifted out of the visible range at one end of the spectrum would simply be replaced by previously invisible rays shifted into it at the other end.Buijs-Ballot was not the only one studying the effect of motion on sound. In an 1848 lecture, French physicist Armand Hippolyte Louis Fizeau announced his own conclusions on how the motion of a sound’s source affects what an observer hears. Fizeau was unaware of Doppler’s and Buijs-Ballot’s work, giving him a fresh perspective on how his acoustic experiments might apply to light. He felt sure that stars could and did move fast enough to change the perceived frequency of their emitted light. And although Fizeau doubted such a change would affect a star’s overall color, he suggested it could be detected spectroscopically, thanks to the dark lines Joseph von Fraunhofer had mapped in solar and stellar spectra. He proposed using these lines as benchmarks, like the monotones he had relied on to hear a shift in pitch during his acoustic experiments. Fizeau was optimistic that astronomers could and would develop instruments that could precisely measure such displacements.More questions than answers
In 1850, a detailed summary of Fizeau’s 1848 lecture was included in a review of Doppler’s theory in Répertoire d’Optique Moderne, published by French physicist Abbé Moigno. Physicist James Clerk Maxwell often consulted the Répertoire; in 1857, he turned to this valued resource to read up on Fizeau’s efforts to measure the speed of light in different media. As it happens, the pages on which Moigno described those experiments immediately preceded his review of Doppler’s work.
So, it is hard to imagine that Maxwell was unaware of either Doppler’s ideas or Fizeau’s 1848 lecture by the time he attended a Royal Society meeting on May 26, 1864. At this meeting, Huggins and chemist William Allen Miller read their first joint paper, “On the Spectra of Some of the Fixed Stars,” confirming that star spectra are interrupted by an assortment of dark lines.Their results must have resonated with Maxwell’s thoughts that evening. Inspired by Fizeau’s experiments, he had recently tried to detect Earth’s motion through the luminiferous ether, an omnipresent medium once proposed to explain light’s propagation through empty space. So with dark lines and Fizeau very much on his mind, Maxwell would have listened with keen interest as Huggins and Miller introduced their groundbreaking work on stellar spectroscopy.In his account of the meeting for The Reader, Norman Lockyer, the magazine’s science editor, reported that the two collaborators presented “pretty certain proof of the idea which has long been floating in many minds as to the cause of the colours of the stars,” namely, that they depend “upon the differences of . . . their chemical constitution.” For Lockyer, this meant “that the colours of the stars must be better watched than they have been,” and that “some other theory than Doppler’s must be found to account for their variability.”Maxwell echoed Lockyer’s doubts concerning Doppler’s theory. Using words that sounded like they had come directly from Fizeau’s 1848 lecture, Maxwell is quoted in Lockyer’s article stating that “if the colours were really tinged in consequence of the motion either of the star or our earth, the lines in the spectrum of the star would not be coincident with the bands of the metal observed on the earth, which gives rise to them.”Lockyer ultimately declared that “Messrs. Huggins and Miller, doubtless will not let the matter rest.” And he was right, sort of. Nearly four years later, in April 1868, Huggins submitted a paper to the Royal Society, not to introduce a new theory of star color, but to describe a method to detect and measure the relative motion of Earth and stars along the line of sight, based on Doppler’s principle.The paper was sent to Astronomer Royal, George Airy, for review. He praised Huggins’s detailed account of his instruments and challenges. However, Airy questioned the legitimacy of assuming that the line “at or near F observed in Sirius” was, in fact, due to hydrogen. Airy thought it “illogical” to insist that the line was due to hydrogen because it coincided with the terrestrial hydrogen line, while at the same time arguing that the star was in motion because of its lack of coincidence. Despite these concerns, he pronounced the paper a “very important one,” and recommended that it be published in the Royal Society’s journal of record, Philosophical Transactions of the Royal Society.
Spectroscopy finds its place
The physical theory behind Huggins’ line-of-sight measures was a challenge to the best of contemporary astronomers. A few years later, Airy wrote to physicist George Stokes: “In some of the German pamphlets now afloat, on optical subjects, there is repeated allusion to das Doppelsche Princip, or some such term, conveying the idea that a man named Doppel has introduced some optical principle. It has something to do with change in the velocity of light but I see no clear description of it — can you help me?”Stokes replied by citing Huggins’ 1868 paper — the very paper Airy had himself refereed!Although Airy was acquainted with stellar spectroscopy, such observations did not fit into the mission or rhythms of daily operation of Greenwich Observatory, under his direction. Professional astronomers had little to no interest in the chemical composition of stars. Their job was to observe, map, and track their positions. As they recognized the spectroscope’s power to reveal stellar motion in three-dimensional space, they became persuaded it could contribute in useful ways to their all-important sky-mapping enterprise. By 1876, even Airy had granted the spectroscope a permanent, though limited, place in the Greenwich routine.