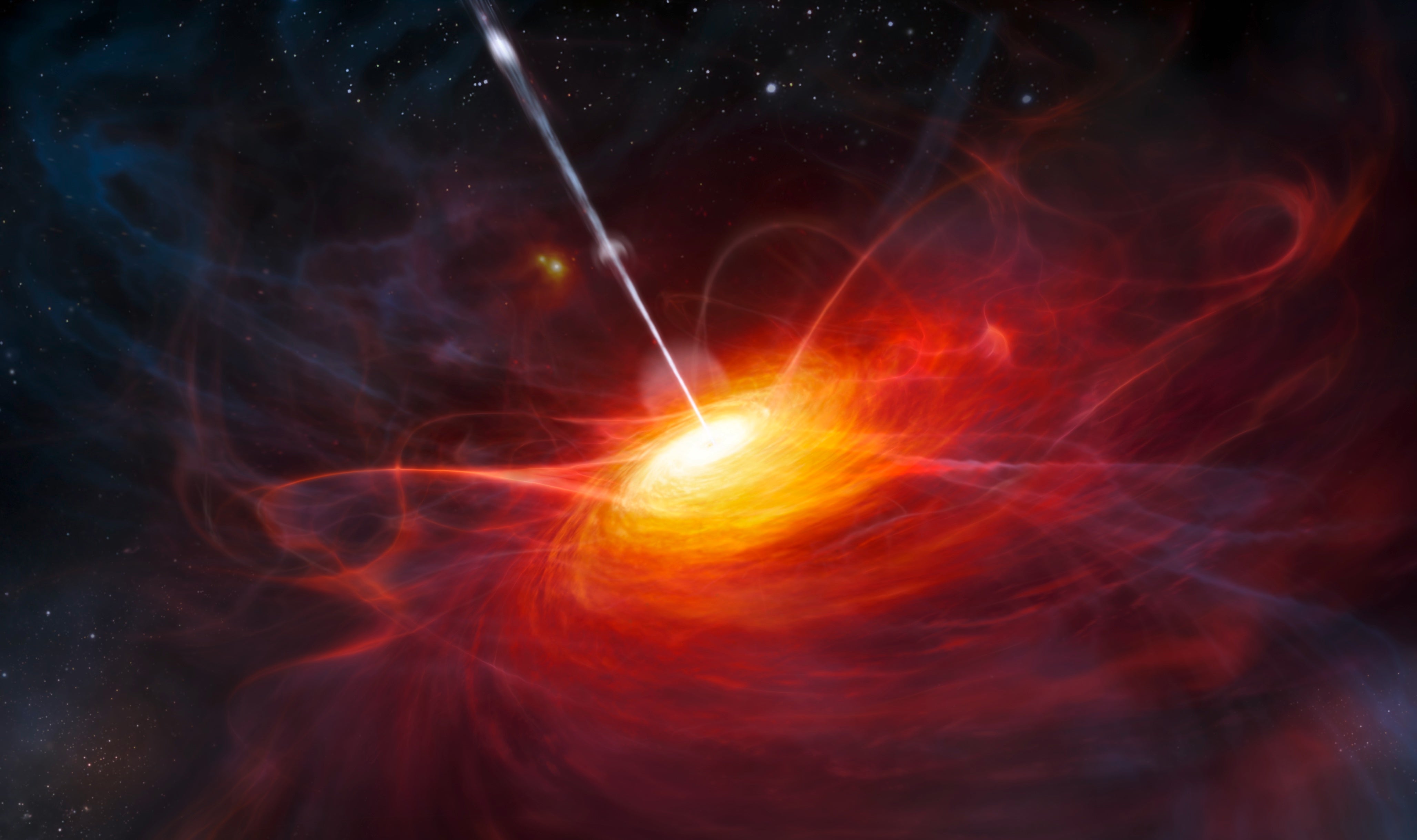
The 1960s were a time of revolution. Antiwar rallies, civil rights marches, and political assassinations dominated the evening news, while radical advances in spaceflight culminated with the first humans landing on the Moon in July 1969.
Astronomy experienced its own ’60s revolution, as a trio of totally unexpected discoveries rocked the science world. In 1964, radio astronomers Arno Penzias and Robert Wilson discovered the fading afterglow of the Big Bang with a horn-shaped antenna at Holmdel, New Jersey, revealing the cosmic microwave background radiation. Then in 1967, Jocelyn Bell, working at the Mullard Radio Astronomy Observatory in Cambridge, England, detected the first pulsars — rapidly rotating neutron stars that some supernovae leave behind.
But before those two breakthroughs, quasars burst onto the scene in 1963. These strange objects looked like stars yet emitted more energy than entire galaxies. In the six decades since their discovery, astronomers have come to understand they are powered by supermassive black holes at the centers of galaxies. No longer mere curiosities, quasars serve as valuable probes, helping astronomers understand galaxy formation and evolution, as well as the earliest epochs of the universe.
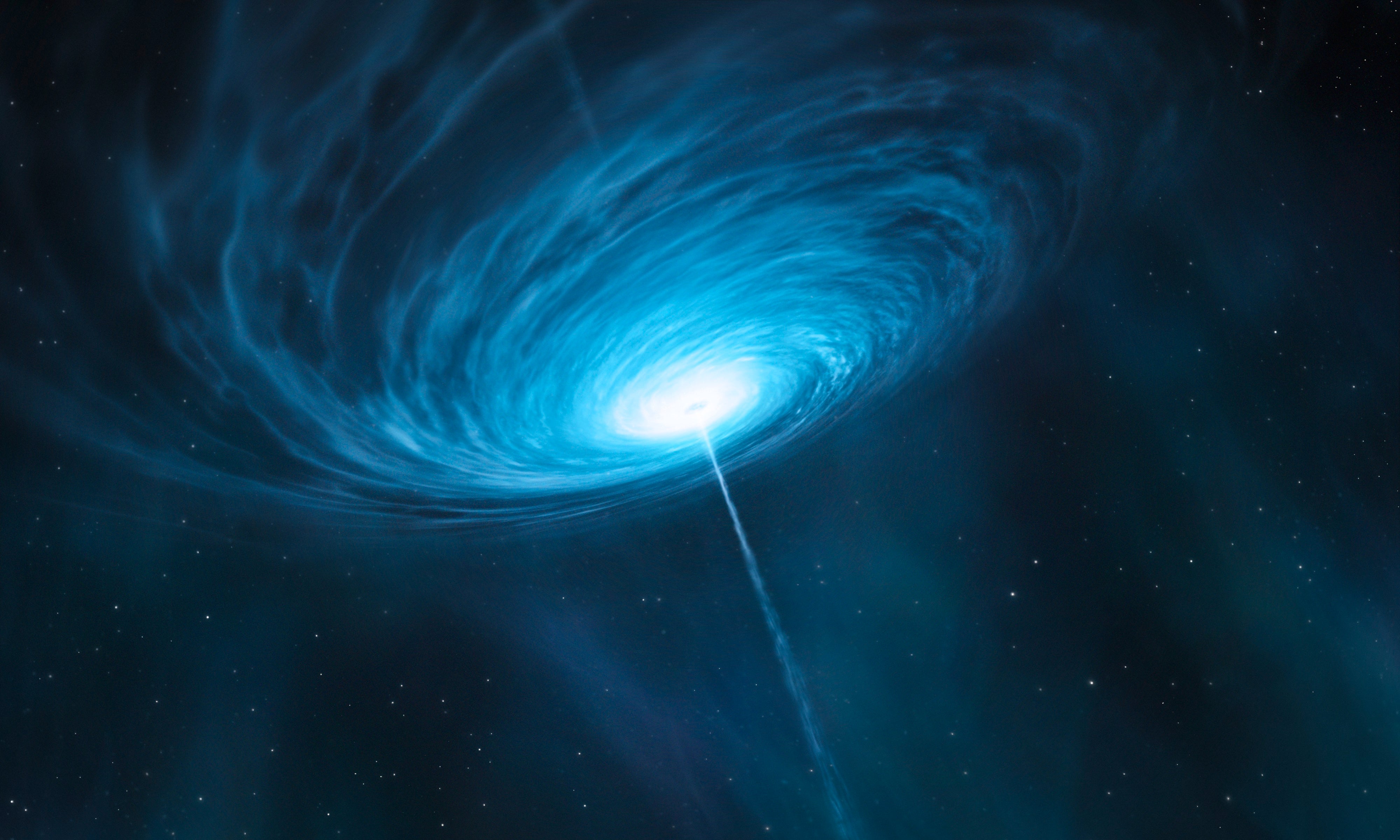
Deep-space beacons
The first hints of quasars came in 1959, when the Third Cambridge (3C) Catalog of radio sources was released, featuring several oddball objects. The strangest were strong radio emitters that looked like stars; they were dubbed quasi-stellar radio sources. Normal stars, like the Sun, glow so dimly at radio wavelengths that researchers never expected to uncover radio-loud stars.
The mystery’s resolution came from the catalog’s 273rd entry: 3C 273. The Moon occulted this object several times in 1962, allowing radio astronomers to pinpoint its location. Caltech astronomer Maarten Schmidt targeted the source with the 200-inch
Hale Telescope, then the world’s largest, and found what seemed to be an unremarkable 13th-magnitude star. But its spectrum was extraordinary. It showed a series of strong emission lines that didn’t seem to correspond to any known element.
Schmidt’s eureka moment came on Feb. 5, 1963, when he recognized the oddball lines as the spectral fingerprint of hydrogen — the universe’s most common element — shifted from its normal wavelengths far to the red portion of the spectrum. This redshift implied 3C 273 was receding from Earth at nearly 16 percent the speed of light!
No star behaves like this. Schmidt quickly realized that 3C 273’s redshift derived from the expansion of the universe, placing the object some 2 billion light-years from Earth. To appear as a 13th-magnitude object — bright enough to show up in modest amateur telescopes (see page 24) — 3C 273 must have a luminosity 40 times that of the brightest galaxies known at the time.
Schmidt and his Caltech colleague Jesse Greenstein immediately recognized that another quasi-stellar radio source, 3C 48, had an even higher redshift and lies well beyond 3C 273. A year later, Taiwanese-American astrophysicist Hong-Yee Chiu shortened “quasi-stellar radio source” to “quasar,” and the name stuck.
In the 60 years since, researchers have cataloged more than a million quasars. Oddly, only about 10 percent emit radio waves, but these radio-quiet quasars otherwise mimic their louder siblings.
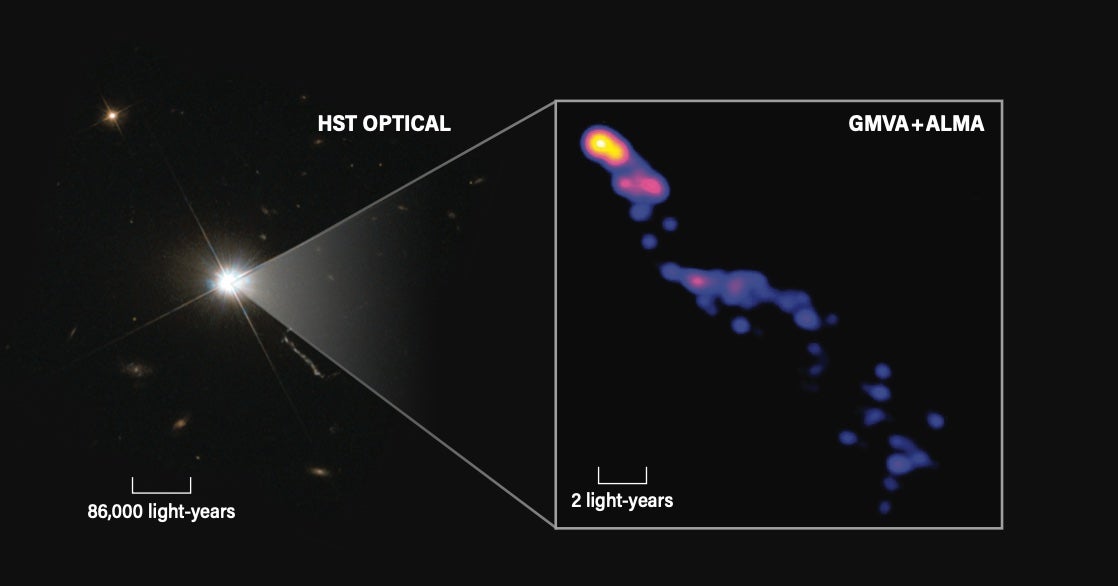
From darkness, light
It quickly became apparent that quasars must tap into a previously unknown energy source. Not only do quasars appear starlike, but they also change brightness in periods as short as a day. Because no object can vary in less time than it takes light to cross it, this means some quasars are no bigger than a light-day across, or just a few times wider than Neptune’s orbit around the Sun. And quasars radiate far more light from this tiny volume than entire galaxies.
Nuclear fusion, which powers the Sun and other stars, converts less than 1 percent of a star’s mass into energy and can’t come close to the power quasars emit. The answer seemed incredible at the time: supermassive black holes containing millions or billions of times the Sun’s mass at the centers of galaxies, actively feeding on surrounding stars and gas.
Although suggested in the mid-’60s, the idea didn’t gain wide acceptance for a couple of decades because many scientists considered black holes purely theoretical. But astronomers now think that depending on the black hole’s rotation rate, this process can convert up to 42 percent of the material’s mass into electromagnetic energy.
A black hole’s gravity is so strong that it can tear apart a star or gas cloud that ventures close. As the stripped material spirals toward the black hole’s event horizon — the point of no return at which nothing, not even light, can travel fast enough to escape — it forms a flattened accretion disk. The gas swirls around at speeds approaching that of light. Friction among the atoms heats the gas so much that it produces the intense radiation we see.
Closer to the black hole, where the atoms move faster and temperatures rise higher, the accretion disk emits mostly ultraviolet light. As temperatures dip farther out, the radiation comes out at lower-energy visible and infrared wavelengths. Most of the X-rays seem to emanate from a much larger corona of gas that envelops the disk. According to quasar expert William Keel of the University of Alabama, “Narrow [optical] emission lines come from low-density gas as it absorbs the ionizing ultraviolet light from the inner accretion disk and electrons recombine with the ions, rattling around and exciting additional ions by collisions along the way.”
Accretion disks often amplify existing magnetic fields. “Around a black hole, the field lines passing through the accretion disk wind around as it rotates, creating a tunnel stretching away from the black hole perpendicular to the disk,” says astrophysicist Belinda Wilkes, a visiting fellow in the School of Physics at the University of Bristol, U.K. “Charged particles trapped by the magnetic field move very fast along the field lines away from the black hole, forming bipolar jets that grow larger than the galaxy itself. The particles emit radiation in the radio, X-ray, and sometimes visible light parts of the electromagnetic spectrum.”
We do not observe the quasar’s electromagnetic radiation at the wavelengths it is initially emitted — cosmic expansion shifts them to the red. For relatively nearby quasars, the difference is dramatic but not extraordinary. For example, the lines 3C 273 emits appear 16 percent longer at Earth than at the quasar, reflecting the object’s redshift of 0.16.
Most quasars lie much farther away. Their population peaks at redshifts between 2 and 3, meaning the observed wavelengths appear three to four times longer than the emitted wavelengths. This corresponds to objects that existed some 3 billion to 2 billion years after the Big Bang and whose light has been traveling toward Earth for 10 billion to 11 billion years.
Giving feedback
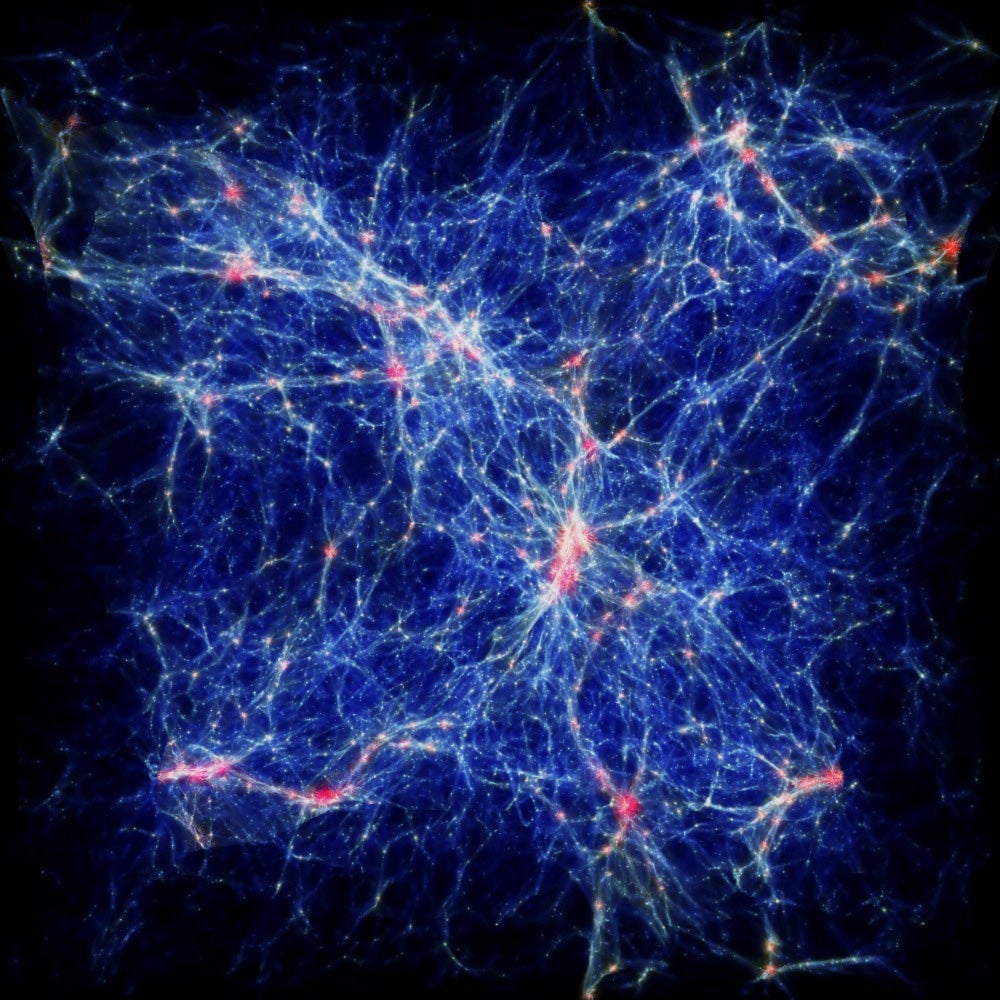
In a laboratory on Earth, an electron’s transition from one energy level to a lower one results in emission of a single photon at a precise wavelength. Nature proves messier. Observed emission lines spread out because enormous numbers of atoms radiate at the same time, and these atoms move with respect to Earth. The Doppler effect causes a photon emitted by material moving toward you to shift to a shorter wavelength (bluer light), while material moving away delivers light of longer wavelengths (redder light).
The smeared emission lines from a quasar’s accretion disk thus carry vital information: The lines’ widths reveal how fast the gas rotates around the black hole. And once astronomers know how long it takes the material to orbit the central black hole, they can calculate its mass.
One of the key discoveries in the early years of the 21st century is that most large galaxies possess central supermassive black holes. Even more intriguing, the mass of the black hole seems to correlate with the mass of the host galaxy’s bulge — even though the bulge typically outweighs the black hole by a factor of 500 to 1,000.
Scientists find such correlations compelling evidence that the growth of central black holes influences the growth of galaxies. “Most people think they both grow because they tap into common reservoirs of matter — the interstellar gas in a galaxy,” says Keel. “As gas enters the galaxy and cools, some forms stars and some, less directly, falls close enough to the core to feed the supermassive black hole.”
But the black hole doesn’t swallow everything. “Although some of the infalling matter falls into the black hole and feeds its growth and activity, the rest streams out along the jets back into and through the galaxy,” says Wilkes. “This combination of inflow and outflow connects the evolution of the black hole with that of its host galaxy in a feedback loop.”
The connection between galaxy and black hole only grows over time. “Galaxy mergers are common and imply that the black holes will eventually merge as well,” says Keel, meaning that the masses of both galaxies and their black holes will grow. So even if there is no relationship between the mass of a black hole and its galaxy to begin with, as they merge with others many times over, a correlation will emerge.
Lighting the cosmos
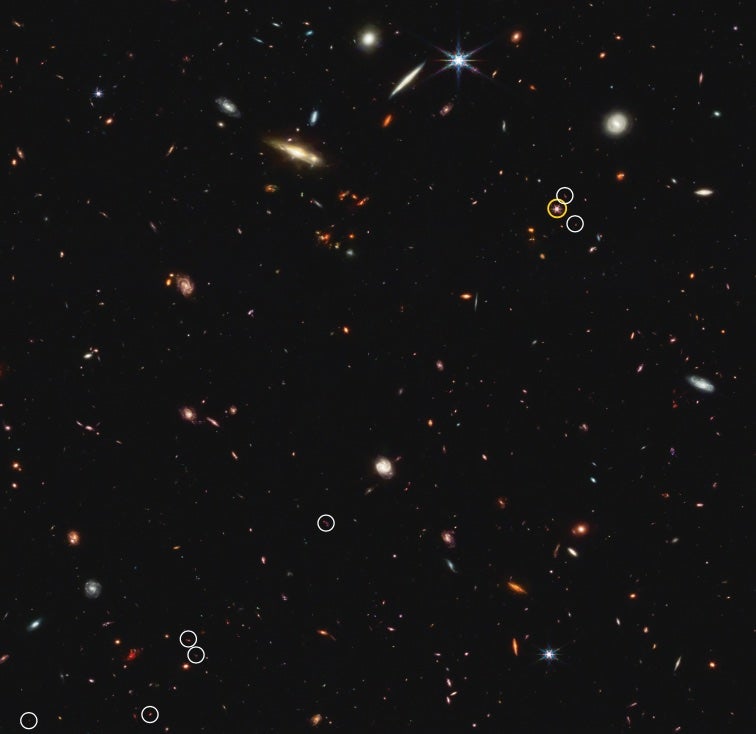
With the exceptions of fleeting events like supernovae and gamma-ray bursts, quasars are the brightest objects in the universe. This makes them powerful probes of the distant universe. And because photons from these objects take so long to reach us, they also shed light on conditions when the cosmos was younger.
Once astronomers started finding quasars with redshifts greater than 2, they noticed a new characteristic in their spectra: Just next to the quasar’s Lyman-alpha (Lyman-α) emission line stood a series of narrow absorption lines, extending to shorter wavelengths. (Lyman-α emission comes from hydrogen atoms whose electron falls to the ground state from the next highest energy level; it has a wavelength in the ultraviolet of 122 nanometers.) In 1971, Roger Lynds deduced that these absorption features, dubbed the Lyman-α forest, arise from cold hydrogen gas in foreground objects that absorb the quasar’s Lyman-α emission. Because these objects lie a bit closer to Earth they have smaller redshifts, so the lines appear at slightly bluer (shorter) wavelengths.
Each “tree” in the forest corresponds to a separate cloud of intergalactic hydrogen lurking in a foreground galaxy cluster. The fact that the trees grow more numerous closer to the quasar means the early universe possessed more galaxy-building material. Recently, astronomers have found similar forests attached to emission lines of heavier elements, revealing that the infant cosmos wasn’t just a pristine mix of hydrogen and helium.
Pushing the limits
To extend the boundaries of our understanding to the edge of the observable universe, astronomers need the power of the James Webb Space Telescope (JWST). Its 6.5-meter mirror gathers more light than most ground-based telescopes, and its sensitivity to near-infrared light makes it ideal for viewing galaxies and quasars at the highest redshifts.
University of Arizona astronomer Feige Wang studies the most distant quasars and their environments, particularly those with redshifts greater than 5. Scientists so far have found about 1,000 quasars at these distances, but only 200 lie beyond redshifts of 6 and just a handful exist at redshifts of 7 or more.
Wang and his colleagues are observing 25 distant quasars with the new space telescope, using a mode that allows them to measure the redshift not only of the quasars but also all surrounding objects in the field of view.
In late June, the team announced their initial results. The first quasar in their study hosts a dark matter halo on the order of a trillion solar masses and anchors a 3-million-light-year-long filament of 10 galaxies. “This is one of the most extended and richest protoclusters yet known in the early universe,” says team member Xiaohui Fan of the University of Arizona. “It shows that luminous quasars powered by supermassive black holes (in this case about 1 billion solar masses) are at the center of an emerging large-scale structure.” Fan adds that if we could see this region as it exists today, the protocluster would likely have evolved into a rich galaxy cluster.
In a second study, the astronomers measured the sizes of the supermassive black holes in eight quasars, all of which have redshifts around 6.7, so we see them as they were some 800 million years after the Big Bang. All eight tip the scale at about a billion solar masses. “We also observed strong winds powered by the central black holes that could extend to the scale of quasar host galaxies,” says Wang. “This provides observational evidence of the existence of quasar feedback in the early universe.”
Of course, eight is a small sample size. Wang says that future JWST observations of a large number of supermassive black holes will provide statistical information about “the general properties of the earliest black holes and help us to understand the interplay between black holes and their host galaxies.”
The findings are changing how astronomers think about galaxy evolution, including the question of which came first: the galaxies or the supermassive black holes at their centers. When astronomers first uncovered the relation between galaxy and supermassive black hole masses, “it seemed crazy to think the black holes came first,” says Keel. “But seeing some quasars with billions of solar masses in the early universe, even if they weren’t first, they must have gotten a head start on growth compared to the surrounding galaxies.”
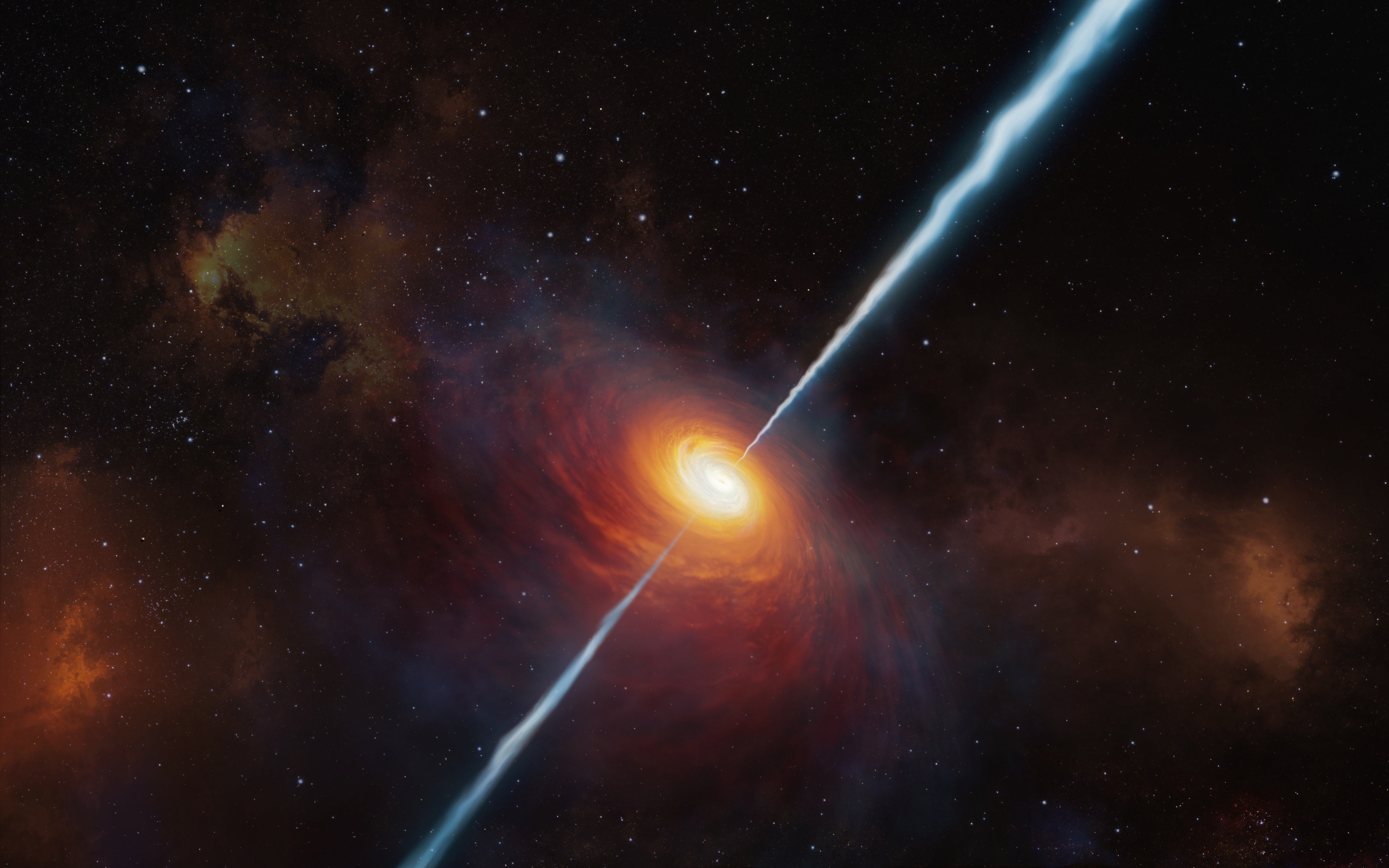
The future beckons
JWST’s time may be precious, but that won’t keep astronomers from using it to further explore quasars and the nascent universe. The early results have been tantalizing, and the relationships between the major players in the cosmos’ first billion years are starting to come into focus.
“The connection is that in the early universe, the most luminous quasars are powered by the most massive black holes, in the most massive galaxies, and residing at the centers of the largest structures with the most active galaxy formation,” says Fan. “So, from black hole to galaxy to the cosmic web, they are all part of the same cosmic ecosystem.”
The findings seem consistent with the apparent coevolution between supermassive black holes and galaxies in the more mature universe. Perhaps the biggest mystery to solve will be finding out how such large structures managed to form so quickly after the Big Bang. (See “Too big, too soon” in the September 2023 issue.)
Fan sees a future where JWST not only investigates the roles of black hole and quasar creation in the context of galaxy formation, but also probes how quasars anchor the universe’s earliest large-scale structures. Keel foresees quasars continuing their yeoman work as beacons lighting up the intergalactic medium and revealing its composition.
Whatever the future may bring, it seems certain quasars will keep astronomers busy for another 60 years.